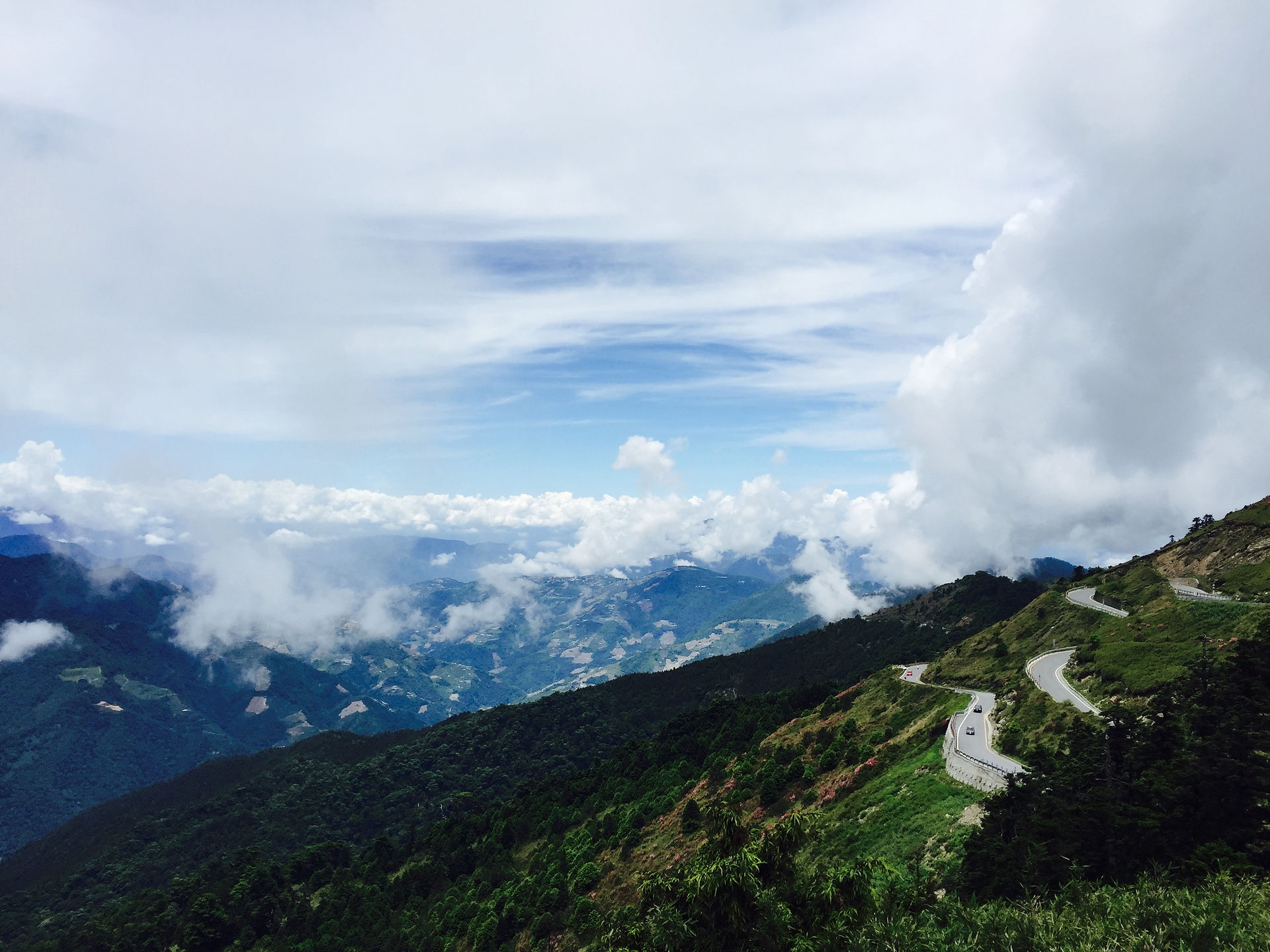

Yuki Haba
The Evolution and Genomics of Behavior
Questions
Current Research
Why and how behavior evolves: the genomics and neurobiology of behavioral evolution in nature
-
What is the genetic architecture underlying behavioral evolution?
-
Which and how many genes / neurons are involved in behavioral evolution?
-
Are there any general principles of genetic/neuronal evolution underpinning behavioral evolution?
The behavioral evolution of mosquitoes

For my PhD project at Princeton University, I study the genomics and neurobiology underlying the evolution of behavior in Culex pipiens mosquitoes, supervised by Dr. Lindy McBride.
Mosquitoes are the most deadly animal in the world.
By transmitting devastating infectious diseases, mosquitoes kill more than a million people annually all over the world.
Importantly, the unique behavioral evolution in mosquitoes, namely the evolution of innate preference toward humans, makes them the most efficient and deadly vectors of infectious diseases.
However, we know next to nothing about the mechanistic basis of their innate preference: Which and how many genes are involved the evolution of preference? When and how many times have the evolution happened? What neural changes are driving the behavior toward humans?
Those are fascinating evolutionary questions per se, but knowing how and why mosquitoes started to like humans would inform the public health effort to prevent transmission of infectious diseases.
We are taking genomic and neurobiological approaches to understand how and why mosquitoes evolved their preference.
This study is supported by:
PacVec CoE Training Grant & Masason Foundation Research Grant.
Culex pipiens. PC: Ary Farajollahi
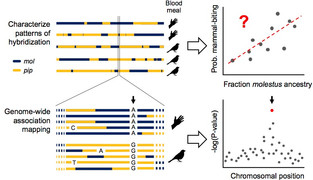
The genomics of local behavioral adaptation
For my MA project, I have been studying genomic signetures of adaptation in wild populations of an Asian beetle, supervised by Dr. Dustin Rubenstein and Dr. Sheng-Feng Shen.
*This study was awarded the 2017 Alfred Russell Wallace Prize for the best MA thesis at Columbia University!
Nicrophorus nepalensis, a species of burying beetle living across Asian countires, shows a variety of behaviors depending on different pressures of competition with flies over resources – specifically, a carcass.
There are two populations of N. nepalensis in Taiwan that differ in breeding season. In the northern part of Taiwan, N. nepalensis can bury a carcass and feed their offspring with it only in winter, whereas in central Taiwan they breed in all seasons.
This difference is attributable to different strategies under competitive conditions; in the central population, they move up in elevation on high mountains to lessen competition with flies that are strong competitors at high temperatures. Furthermore, in the summer at low elevations, burying beetles tend to cooperate in order to bury a carcass swiftly before flies find it. In contrast, in the northern part of Taiwan, where mountains are not high enough, the activity of flies is so intense that beetles cannot outcompete them even by cooperatively burying a carcass. As a result, they do not breed until winter comes, when fly activity decreases.
We are now working to find genetic/genomic signatures underlying these local adaptations; we have sequenced whole genomes from both populations and are conducting RNA-seq.
We are also interested in ecological consequences of different behavioral strategies in each environment.

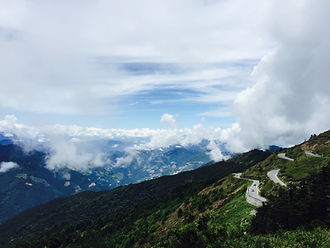
Past Research
A novel PCM to analyse complex evolutionary scenarios
With Dr. Nobuyuki Kutsukake, I developed a novel phylogenetic comparative method to analyse data where continuous and discrete traits are biologically interwoven.
In evolutionary studies analysing interspecific variation of phenotypic traits, data often include both discrete and continuous variables, interrelated with each other. In such cases, an analysis of either variable alone will miss complex features of biological phenomena.
We developed a novel phylogenetic comparative method (PCM) that enabled analysis of inter-related discrete and continuous variables within a single framework. This framework uses a PCM with approximate Bayesian computation (ABC). Therein, trait simulations on a phylogeny can incorporate various types of link functions such as non-linear relationships between discrete and continuous traits.
As an example, we analysed interspecific variation in group size (continuous variable) among 10 species of African subterranean rodents that have social systems (discrete variable) ranging from solitary to eusocial. We could estimate (1) the evolutionary probability functions that link social states and group size, (2) the ancestral state, transition processes of sociality, and group size at each node, and (3) the presence/absence of directional selection on the branches leading to eusocial species.
This study is the first to provide a framework for analysing evolution of continuous and discrete traits interrelated in a complex manner, and it also demonstrates the flexibility of the simulation-based approach in PCMs.
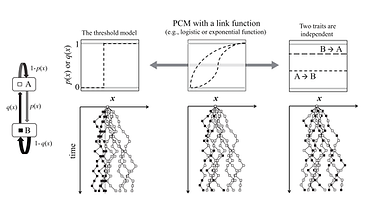
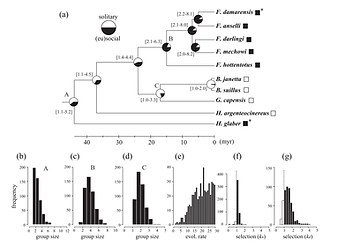


Evolution of PRDM9 gene across vertebrates
At Dr. Molly Przeworski's lab, led by Zach Baker and Dr. Molly Schumer, I have participated in the project to understand the evolutionary trajectories of PRDM9 gene.
Studies of a handful of species reveal two mechanisms by which meiotic recombination is directed to the genome - through PRDM9 binding or by targeting promoter-like features - that lead to dramatically different evolutionary dynamics of hotspots.
Here, we identified PRDM9 from genome and transcriptome data in 225 species, finding the complete PRDM9 ortholog across distantly related vertebrates. Yet, despite its broad conservation, we inferred a minimum of six partial and three complete losses.
Strikingly, taxa carrying the complete ortholog of PRDM9 are precisely those with rapid evolution of its predicted binding affinity, suggesting that all its domains are necessary for directing recombination. Indeed, as we show, swordtail fish carrying a partial ortholog share recombination properties with PRDM9 knock-outs.
